The growing imperative to use
energy as efficiently as possible, combined with the need to create
electricity from a wider variety of energy sources including renewables,
is driving growing interest in DC power distribution over long
distances and within buildings.
AC vs DC Distribution: Conflict and Coexistence
Nikola Tesla’s victory over Thomas Edison in the arguments that led the world’s power grids to adopt high-voltage AC distribution could be short-lived: after only about 130 years, the technological pendulum could be swinging back in favor of high-voltage DC (HVDC) distribution.As things stood in the late 1880s, AC power distribution was considered a better economical and practical proposition, despite some imperfections. Long-distance power transmission occurs at high voltages to minimize I2R losses, and at the time the required step-up was easier to achieve using AC-operated transformers than with the rotating machines needed to generate high DC voltages. Even at the time, suitable transformers were also smaller, less expensive and more reliable.
The arrival of mercury-based rectifiers in the early 20th century, later followed by high-voltage, high-current solid-state thyristors and most recently IGBT modules (Figure 1), generation of high DC voltages became simpler thereby making the advantages of HVDC distribution more accessible and attractive.
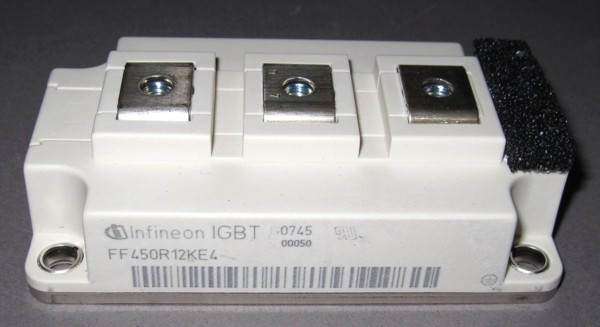
Figure 1: Modern power semiconductors such as high-voltage, high-current IGBT modules enhance the economics and reliability of HVDC transmission. Image courtesy of Infineon.
HVDC can overcome some of the drawbacks encountered when distributing power at high AC voltages. HVDC transmission distances and capacity are not limited by inductive and capacitive effects such as charging currents. In addition, the entire cable cross section can be utilized to support current flow, as there is no skin effect. Moreover, AC power does not permit transmitting power between networks operating at different frequencies, or where the networks cannot be synchronized. In addition, concerns over instability or unsuitable power flows can prevent making new connections.
Today, HVDC is alive and well in numerous distribution projects worldwide, such as long-distance links like the Caprivi Link in Namibia and the Three Gorges-Guangdong link in China that are each over 900 kilometers, as well as various cross-border and cross-sea links throughout Europe, New Zealand, the Japanese islands and elsewhere. Back-to-back HVDC connections are also widely installed as a stable and convenient means of sharing power between AC grids.
HVDC in the Grids of Tomorrow
HVDC distribution could become even more pervasive as environmentally-driven changes in power-generation policy drive progress toward distributed power generation supplying hybrid infrastructures that comprise diverse types of grids known collectively as the Smart Grid.Power generation is moving from a centralized model reliant on a small number of large fossil-fuel power stations to incorporate a variety of sources, such as energy generated by wind or solar farms. The raw output from a wind turbine has unstable voltage and frequency, and so is typically converted to DC to facilitate stabilization, before being re-converted to the required AC frequency and voltage for feed-in to the grid. Similarly, the DC output of a PV array must be converted to AC of the right voltage and frequency before it can be put on the grid.
With the increased reliance on non-fossil-fuel energy sources, generation is becoming more distributed as solar and wind farms are installed in naturally advantageous locations and small local wind or solar generators – microgenerators – are permitted on commercial or residential premises. These do not have to be connected to the grid, although generous feed-in tariffs are sometimes offered to encourage owners to sell any unused capacity to their utility company.
As power generation is moving towards a more distributed model with privately owned microgenerators, whether connected to the main grid or otherwise, the concept of the microgrid is emerging (Figure 2). A microgrid combines localized groups of electricity sources and loads that can be controlled and managed either as islands or when synchronized and connected with the main power infrastructure, or macrogrid. Microgrids can potentially deliver several advantages, including reduction in greenhouse gas emissions, lower utility bills for end users, and a means of sharing the cost of modernizing or upgrading local power infrastructures. Microgrids exist within the concept of the Smart Grid, and can help to realize its key objectives, such as stability, reliability, and security of electricity supply as energy sources become more distributed and less predictable.
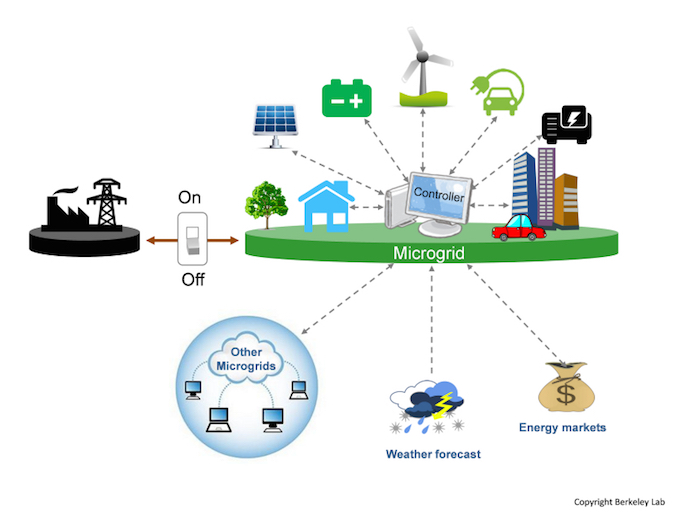
Figure 2: A microgrid can be powered from various sources, and connected to the main grid or islanded. Image courtesy of Berkeley Lab,
Changing Consumption Argues for DC Microgrids
Alongside the changes taking place in the distribution infrastructure, the power needs of electricity users have also changed significantly since the early decisions regarding electricity distribution were made. Not only has overall power demand risen steadily, but the nature of the equipment being used in typical homes and businesses has also changed.For much of the second half of the 20th century, electricity in even the most state-of-the-art homes was consumed predominantly by AC-powered incandescent lighting and a small number of appliances such as washing machines, refrigerators and dishwashers containing large AC induction motors. In contrast, in today’s homes, there is a shift towards LED lighting, which is an inherently DC-powered technology, as well as new and more energy-efficient appliances that contain variable-speed drives featuring smaller and sometimes brushed or brushless DC motors. There are also now large numbers of electronic devices such as PCs, routers, game consoles, and set-top boxes that operate from an internal or external AC/DC power supply. Many other devices, such as tablets, smartphones or cordless power tools, although battery-powered, are recharged at low DC voltages. Today’s homes contain more electrical and electronic devices than ever before, each containing its own circuitry to convert the standard high-voltage AC-line supply to a suitable low DC voltage at the point of use.
Residential homes are not the only premises whose electricity consumption is dominated by essentially low-voltage DC-powered equipment. The telecom switches and data centers at the heart of today’s digital economy are among the largest consumers of power in the modern world. One data center can consume as much energy as many thousands of homes, although the goal is to power servers that require DC rails at voltages down to 1V or less.
In the never-ending search to maximize efficiency and eliminate as far as possible any energy losses, the losses incurred during power conversion, between AC and DC as well as between different DC voltages, is coming under increased scrutiny. Some within the data center industry are advocating HVDC distribution as the most efficient approach for distributing power within the premises.
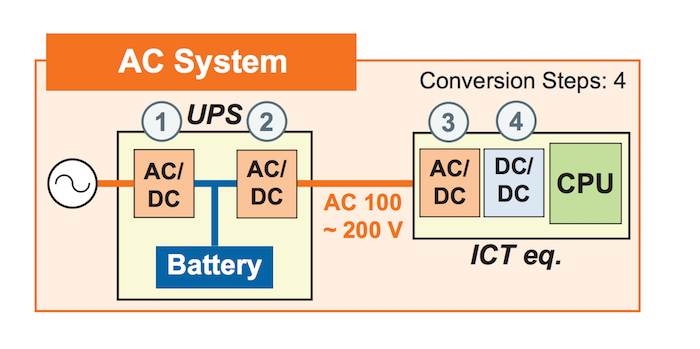
Figure 3: Conventional AC distribution in data centers involves multiple conversion steps. Image courtesy of Vicor
A conventional strategy for data center power distribution (Figure 3) initially steps-down and rectifies the incoming AC line supply to allow interconnection with a battery backup system. The output of this network, which is often 48V DC, is then converted to an AC voltage of about 200V for distribution within the building. At the cabinet level, this high-voltage AC supply is rectified and down-converted to an intermediate DC voltage and ultimately converted at the point of load to provide the desired power rails for the processors and other ICs on the server boards. Alternatively, the output from the high-voltage AC/DC power supply and battery backup network, typically at 48V DC, is distributed throughout the data center and then converted by a combination of intermediate and point-of-load converters on the server boards.
As data center power demands continue to rise, concern is growing over the energy lost through multiple power-conversion stages. In 48V DC systems, I2R losses are considerable when supplying the latest servers that can consume well over 1kW at peak power. While discussion continues about how to make AC distribution systems more efficient, HVDC distribution at a voltage of 380-400V promises a way of reducing I2R losses while also eliminating the DC-to-AC inverter and its associated losses. The incoming AC supply at line voltage is rectified and converted to a high DC voltage of 380-400V. Large amounts of power can be distributed at this high voltage at very low current, resulting in minimal energy loss. The high voltage is then stepped down using straightforward DC-to-DC conversion in the cabinets. HVDC architectures have been proposed that can be adopted incrementally in data centers that are currently either using high-voltage AC or low-voltage DC distribution.
This trend within the data center industry is strengthening the case in favor of DC power distribution within buildings. The EMerge Alliance is championing the cause of DC distribution in commercial buildings, and developing standards that aim to enable hybrid AC and DC infrastructures to maximize flexibility as well as efficient use of energy and space. The Alliance has published standards for 24V DC distribution within occupied spaces, and for hybrid AC and 380V DC distribution in data centers (Figure 4) and telecom offices.
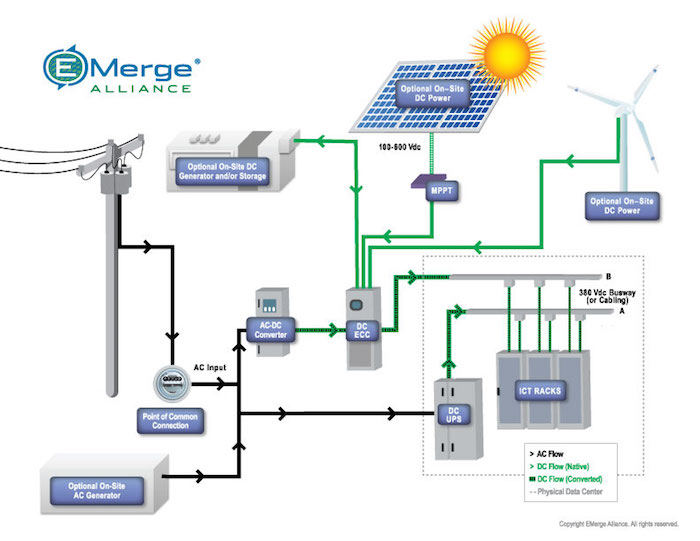
Figure 4: The Emerge Alliance has developed standards for high-voltage DC distribution in data centers. Image courtesy of EMerge Alliance
The 24V DC standard proposes an in-building microgrid for powering loads such as lighting, PCs, projectors or televisions. More than 60 products from well-known manufacturers have so far been registered, including LED lamps, fluorescent ballasts, overhead fans, converters, control units and wiring products. The Alliance is also developing a standard for DC microgrids to supply loads in outdoor spaces such as exterior lighting and electric-vehicle chargers. In addition, 380V DC could be proposed for powering large building loads such as HVAC systems, industrial equipment, and high-bay lighting, as well as domestic appliances such as ovens and washing or drying machines with variable-speed drives.
Conclusion
The contest between AC and DC distribution techniques, often imagined as a battle won by AC at the beginning of the electrical age, has in fact evolved into peaceful coexistence as engineers have developed technologies to take advantage of the strengths of either approach depending on the needs of any given application.DC distribution at very high voltages over long distances, or within localized areas or throughout buildings at voltages such as 380V or 24V, is likely become more prevalent as power-generation policies move to integrate more renewable energy sources and micro-generation, and as end-user demands continue to shift towards low-voltage electronic equipment. This may encourage widespread adoption of DC microgrids, which could, in turn, drive significant redesign of all sorts of equipment from home electronics and PCs, to battery chargers and power adapters as well as wall outlets and light switches.
No comments:
Post a Comment